 |
Climate change output
CSIRO Atmospheric Research Technical Paper No. 37
1998
Kevin J. Hennessy
Contents
Abstract
Background
Global climate models
Regional climate models
CSIRO climate models
Overview
CSIRO Mark 2 global climate model with slab ocean
(CSIRO slab)
CSIRO regional climate model (DARLAM)
CSIRO global coupled ocean-atmosphere-sea-ice model (CSIRO
coupled)
Experiments conducted
Experiment 1: CSIRO Mark 2 slab GCM 1×CO2
and 2×CO2 simulations
Experiment 2: DARLAM 1×CO2 and 2×CO2
simulations
Experiment 3: CSIRO coupled GCM transient CO2
simulation
Output available
Value-added products
Tailored output
OzClim PC software
Licence
agreement
Obtaining output
Appendix 1: CSIRO Mark 2 global climate model output
Appendix 2: CSIRO regional climate model (DARLAM) output
Appendix 3: CSIRO coupled ocean-atmosphere global climate model output
References
Acknowledgments
Abstract
The CSIRO Climate Change Research Program is Australias
largest and most comprehensive program investigating the greenhouse effect
and global climate change. This document lists the output from CSIRO climate
models that have been used to conduct enhanced greenhouse experiments.
Two global climate models (GCMs) and a regional climate model (RCM) are
described.
Three CSIRO enhanced greenhouse experiments were undertaken. Provision
of output from these experiments is intended to give other scientists
an internally consistent set of detailed climatic variables for use in
sensitivity studies. The first experiment was performed in 1994 with the
CSIRO Mark 2 slab GCM, where enhanced greenhouse conditions were represented
by an instantaneous doubling of CO2. Output
from this experiment was fed into the second experiment in 1995 which
involved running a high resolution RCM over Australasia to produce more
detailed information. The third experiment was performed in 1996 with
the CSIRO coupled GCM which was driven by a gradually increasing CO2
concentration scenario for 185 years.
A wide range of climatic variables was saved from each experiment at
various time intervals and at various vertical levels. Broad groupings
of the variables include temperature, precipitation, wind, pressure, cloud,
evaporation, radiation, humidity, soil moisture, runoff, snow, sea-ice,
mixing ratio, and heat flux. Many options exist for adding value to output
saved from these experiments, through manipulating data to suit specific
needs. The PC-based software package called OzClim enables regional scenarios
of climate change to be generated for the whole or selected parts of Australia
at various spatial resolutions, for any date between 1990 and 2100, where
the user can select from a range of greenhouse gas emission scenarios,
global climate sensitivity assumptions, and GCM or RCM patterns of climate
change.
A detailed list of output from each experiment is supplied and steps
required for obtaining output from CSIRO are explained.
Background
The climate model data presented in this report are a product of the
CSIRO Climate Change Research Program (CCRP). The CCRP is Australias
largest and most comprehensive program investigating the greenhouse effect
and global climate change. It involves at least nine CSIRO Divisions and
integrates work from researchers in other research institutes, particularly
the Bureau of Meteorology, the Antarctic
Research Centre, and the Cooperative
Research Centre for Southern Hemisphere Meteorology.
A major component of the CCRP is a project entitled Climate Change
which draws on work from other CCRP projects to form a basis for modelling
climate change. This document lists the output from CSIRO climate models
that have been used to conduct enhanced greenhouse experiments. Two global
climate models (GCMs) and a regional climate model (RCM) were used.
Global climate models
A global climate model is a computer model representing the atmosphere,
oceans, land and icecaps. By solving mathematical equations based upon
the laws of physics, a GCM simulates the behaviour of the climate system.
The model divides the planet into a number of vertical layers representing
levels in the atmosphere and depths in the oceans, and divides the surface
of the planet into a grid of horizontal boxes separated by lines similar
to latitudes and longitudes. In this way, the planet is covered by a three-dimensional
grid of boxes (Figure 1).
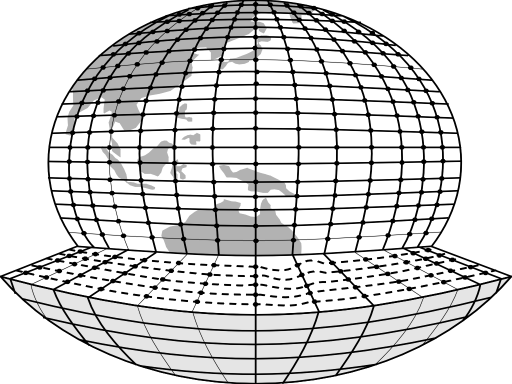
Fig 1. Schematic representation of the grid
of boxes covering the Earths surface and atmosphere in a typical
global climate model.
The horizontal size of a typical grid box in the CSIRO GCM is about 625
km by 350 km, limited largely by computer power. Inside each grid box,
the mathematical equations are solved at model-timesteps of about an hour
for many model-decades until a picture of the Earths climate is
built up. Global climate models capture large scale features like the
deserts and tropics very well, but have difficulty capturing smaller features
like cyclones and thunderstorms because they occur at scales much smaller
than the grid boxes.
Carbon dioxide (CO2) is one of the main greenhouse
gases affected by human activities. A common experiment for comparing
different climate model simulations of enhanced greenhouse conditions
is an instantaneous doubling of the atmospheric carbon dioxide concentration
(2×CO2). The timing of 2×CO2
depends critically on the growth rate of greenhouse gas emissions, and
the rate of uptake of CO2 by the biosphere and
oceans. The Intergovernmental Panel on Climate
Change (IPCC: Houghton et al., 1996) has produced
six emission scenarios which vary widely over the next century. For the
mid-range scenario (IS92a), a doubling of the 1975 CO2
concentration occurs by the year 2100. When the effects of other greenhouse
gases are included, the change in radiation equivalent to a doubling of
CO2 alone occurs by the year 2060 (Dix
and Hunt, 1995).
Over the ocean, most 2×CO2 experiments
use a simple slab of water at the lower boundary which represents
the mixed-layer in the top 50 metres of ocean. Slab ocean experiments
cannot take into account the potential climatic effect of changes in ocean
circulation and the transfer of surface warming into the deep ocean. This
is an important caveat.
Coupled ocean-atmosphere GCMs employ models of the full ocean (including
the deep ocean). They can simulate the uptake of surface warming by the
deep ocean and changes in ocean circulation, and the consequent effect
this has on regional climate change. Some features of climate variability
associated with the El
Niño-Southern Oscillation (ENSO) are also captured by coupled
models. In addition, coupled models are driven by a realistic IPCC scenario
of steadily increasing (transient) concentrations of carbon dioxide when
run under enhanced greenhouse conditions, rather than an instantaneous
doubling of CO2.
Coupled models are conceptually better than models with a slab ocean,
but the choice of model for Australian studies is unfortunately not that
simple. The discussion below outlines why output from both slab and coupled
models should be considered equally valid, at the present time.
In the northern hemisphere, the patterns of simulated temperature and
rainfall change are similar in slab and coupled models. From the perspective
of regional scenario development in the northern hemisphere, the move
to using coupled models is not a big issue. However, the differences are
large in the southern hemisphere. Coupled models simulate a strong uptake
of heat into the deep ocean in high southern latitudes, leading to reduced
surface warming relative to other latitudes, whereas slab models do not
show this reduction in warming. In particular, slab models simulate increased
rainfall over northern and western Australia in summer, but coupled models
simulate decreased rainfall (CSIRO, 1996; Whetton
et al., 1997b).
There are two reasons why coupled models may be over-estimating the reduced
warming in high southern latitudes. Oceanic observations suggest that
the Southern Ocean is not mixed as actively as is typically simulated
in coupled models (England, 1995), and observed temperature
trends this century do not show a reduced warming in higher latitudes
of the southern hemisphere relative to other parts of the world (Kattenberg
et al., 1996). However, slab models may be over-estimating the Southern
Ocean warming because they do not include uptake of heat by the deep ocean.
Therefore, coupled models may be under-estimating the warming in the
Southern Ocean and slab models may be over-estimating the warming. Until
the problems associated with coupled models in the southern hemisphere
are resolved or at least reduced, output derived from both slab and coupled
models are worth analysing for the Australian region.
Regional climate models
To improve regional detail in climate models, it is desirable to reduce
the spacing between gridpoints. However, due to the complexity of global
climate modelling, computational requirements become prohibitive if the
horizontal grid resolution is less than a few hundred kilometres. At this
resolution, vitally important small-scale phenomena, like tropical cyclones
and cold fronts, are poorly captured. This affects simulated patterns
of temperature and rainfall, and hence the ability to realistically simulate
observed regional climate features in GCMs.
A computationally feasible alternative to a coarse resolution global
climate model is to use a finer resolution model over a small part of
the globe. A regional climate model (RCM), with a horizontal resolution
of about 100 km or less, is able to simulate regional weather patterns
better than most GCMs (McGregor et al., 1993). Part of the reason for
the improved climate simulation relative to GCMs is the fact that coastlines
and mountains are represented in more detail in RCMs. Since topographic
features strongly influence regional temperature and rainfall, more detailed
features are likely to give a better climate simulation.
A regional climate model requires weather information at its lateral
boundaries in order to simulate weather within its boundaries. For climate
change studies, an RCM is typically driven at its boundaries by information
from a coarser-scale GCM. This is commonly called nesting an RCM inside
a GCM. One-way nesting allows information to flow from the GCM to the
RCM each simulated day, but the weather simulated by the RCM does not
affect the GCM interactively. This means that the RCM can be run after
the GCM experiment has been completed.
The application of RCMs to decadal-scale climate modelling is only recent,
since RCMs have mainly been used in the past for short-term weather forecasting.
Very few RCMs have been used for climate change experiments, and CSIRO
is a leader in this field. Although the performance of an RCM is constrained
by its reliance on GCM performance at the lateral boundaries, RCMs offer
detailed insight into regional climate change. The ability to use fine
resolution RCM climate change output should be seen as a significant opportunity.
CSIRO climate models
Overview
This section describes the three CSIRO climate models used in enhanced
greenhouse experiments. There are two coarse resolution global climate
models and a fine resolution regional climate model. Since each model
was developed at CSIRO, there are many similarities.
Each model uses the same basic equations which describe the laws of physics.
Schemes for boundary layer mixing, moisture advection, radiation and cloud
formation are also common to each model. The simulation of average climate
for selected variables has been validated against observed average climatic
data as part of standard model testing procedures. Simulations described
in this report are from models which have passed global and Australian
climate validation, so that some confidence may be placed in output from
enhanced greenhouse simulations, taking the following caveats into account.
The models do not (and cannot) take into account all processes (natural
and anthropogenic) which affect climate variability and change. Some processes
are not well understood and others must be represented in a simplified
way in order to ensure computational efficiency. While continental-scale
climatic features are well simulated for present conditions, regional
features are captured with less accuracy.
None of the models includes the regional cooling effect of sulfate aerosol
which has been identified by the IPCC (Houghton et al.,
1996) as an important element of anthropogenic climate change, particularly
in the northern hemisphere where aerosol are emitted in large quantities.
While aerosol emissions in Australia are relatively small, northern hemisphere
aerosol may influence the Australian climate indirectly, through long-distance
climatic teleconnection patterns (e.g. the influence of Asian aerosols
on land-sea temperature gradients which may affect the Australian monsoon).
Simplifications in the representation of ocean processes are likely to
be important in determining patterns of climate change in the Australian
region, such as changes in the vertical profile of ocean temperature/salinity
and the El Niño - Southern Oscillation. The climatic influence
of small-scale features such as tropical cyclones and storms cannot be
resolved at this stage.
Plant physiology is not included, so simulated vegetation does not respond
to climate change or increased levels of CO2.
However, significant biospheric responses to climate change could occur
in the real world, as could changes in land-use, with consequent climatic
feedbacks.
CSIRO Mark 2 global climate model with slab ocean
(CSIRO slab)
The CSIRO Mark 2 GCM is a spectral model with R21 horizontal resolution
(grid boxes measuring about 625 km by 350 km) and has 9 vertical levels
in the atmosphere (Watterson et al., 1997). This
gives 41 grid boxes over Australia. Global atmospheric and biospheric
sub-models are coupled to a slab ocean sub-model. Simplifications of physical
processes such as convection, radiation, gravity wave drag, cloud formation,
sea-ice formation and biospheric interactions are detailed in McGregor
et al. (1993), McGregor (1993), Kowalczyk
et al. (1994) and OFarrell (1998).
Adjusted heat fluxes are applied to the slab ocean to represent heat
from the deep ocean and the effect of currents (Watterson
et al., 1997). Fluxes are determined from a separate 10-year experiment
driven by observed sea-surface temperatures (SSTs). Regional flux adjustments
are required to keep simulated sea-surface temperatures close to those
observed, and these monthly average flux adjustments were saved for use
in 1×CO2 and 2×CO2
experiments. When flux adjustments are applied in the 1×CO2
run, the simulated SSTs and other continental-scale climatic features
are similar to those observed. The same flux adjustments are applied in
the 2×CO2 run, which places an artificial
constraint on the variability of sea-surface temperature as the climate
changes. This limitation may have important implications for projected
ocean behaviour and atmospheric circulation patterns. On a CRAY Y-MP computer,
climate variables for one model day take 30 seconds to evaluate, so a
10 year run takes 50 hours.
CSIRO regional climate model (DARLAM)
Over the Australasian region (71°E177°E, 12°N57°S),
the CSIRO regional climate model (DARLAM) has been driven at its lateral
boundaries by output from the CSIRO Mark 2 GCM (Walsh
and McGregor, 1995; McGregor et al., submitted).
DARLAM has nine vertical levels in the atmosphere and grid boxes measuring
about 125 km by 125 km, giving 442 grid boxes over Australia (Figure
2).
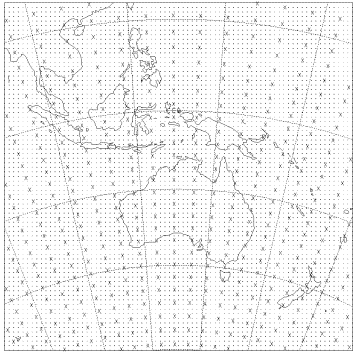
Fig 2. DARLAM model domain for simulations
using a 125 km grid (dots). Gaussian gridpoints of the R21 CSIRO slab
GCM are indicated by crosses (from Walsh and McGregor, 1995).
The atmospheric sub-model interacts with a slab ocean sub-model and uses
descriptions of physical processes which are similar to those in the CSIRO
Mark 2 GCM. However, DARLAM uses a modified convection scheme, a different
soil moisture scheme and excludes gravity wave drag. Sensitivity experiments
showed that results were not greatly affected by the change in convection
scheme. On a CRAY Y-MP computer, climate variables for one model day take
130 seconds to evaluate, so a 10 year run takes 132 hours.
CSIRO global coupled ocean-atmosphere-sea-ice model
(CSIRO coupled)
The CSIRO coupled model involves global atmospheric, oceanic, sea-ice
and biospheric sub-models (Gordon and O'Farrell, 1997;
Hirst et al., 1997). The atmospheric, biospheric and
sea-ice sub-models are the same as those used in the CSIRO Mark 2 GCM.
Atmospheric and oceanic components use a spectral R21 horizontal grid
(each gridbox measuring about 625 km by 350 km) with 9 vertical levels
in the atmosphere and 21 levels in the ocean. The ocean model has a heat
transport scheme which significantly reduces problems associated with
excessive mixing in the Southern Ocean. On a CRAY Y-MP computer, climate
variables for one model day take 60 seconds to evaluate, so a 10 year
run takes 61 hours.
Coupling the atmosphere to the ocean is technically challenging because
the ocean has a much longer timescale of variability than the atmosphere.
The coupled model requires adjustments to the fluxes of heat, salinity
and wind stress which link the atmospheric and oceanic components. Adjusting
the heat fluxes at the ocean/atmosphere/ice interface is performed by
running the ocean and atmosphere models independently and computing (i)
the fluxes required by the ocean model when driven by observed SST, sea-surface
surface salinity (SSS) and wind stress, and (ii) the heat fluxes generated
by the atmosphere/ice model with observed SST and SSS. The flux adjustment
is the difference between (i) and (ii). These adjustments were used in
the fully coupled model which generates its own SST, SSS and wind stress.
The same flux adjustments are applied to the transient CO2
run (Hirst et al., 1997), which places an artificial
constraint on the variability of sea-surface temperature as the climate
changes. Flux adjustments in the coupled experiment are much smaller than
the Q-fluxes in the CSIRO slab experiment.
Experiments conducted
This section describes three CSIRO enhanced greenhouse experiments. Provision
of output from these experiments is intended to give users an internally
consistent set of detailed climatic variables for use in sensitivity studies.
Those wishing to undertake impact assessments or sensitivity studies which
incorporate a wider range of future climates should use the simplified
scenarios of CSIRO (1996) which are based on consensus
results of five international global climate models.
The first experiment described below was performed in 1994 with the CSIRO
Mark 2 slab GCM, where enhanced greenhouse conditions were represented
by an instantaneous doubling of CO2. Output
from this experiment was fed into the second experiment in 1995 which
involved running a high resolution regional climate model over Australasia
to produce more detailed information. The third experiment was performed
in 1996 with the CSIRO coupled GCM which was driven by a gradually increasing
CO2 concentration scenario for 185 years.
Other experiments have been performed and new experiments are planned.
Data sets described below are those which have been checked and published
in peer-reviewed literature. Researchers should ask whether more recent
data sets have been made available since the publication of this document.
More information can be obtained from Dr
Roger Jones.
Experiment 1: CSIRO Mark 2 slab GCM 1×CO2
and 2×CO2 simulations
In the control experiment, the model was run for 30 model-years of 1×CO2
conditions (an atmospheric concentration of 326 parts per million (ppm)
from the year 1973). In the enhanced greenhouse experiment, the model
was run for 55 years of 2×CO2 conditions
(660 ppm), and the global mean temperature reached equilibrium during
the last 30 years. For a doubling of CO2, the
global mean warming at equilibrium is 4.3°C (Figure
3).
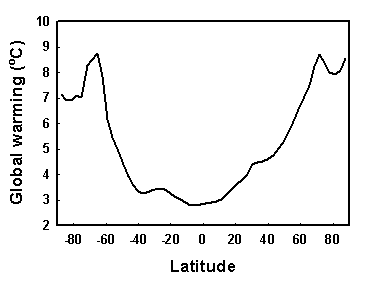
Fig 3. Zonal and annual mean change in surface
temperature for a doubling of CO2 from 330 ppm
to 660 ppm in the CSIRO slab GCM (from Watterson et al., 1997).
The climate variables saved are listed in Appendix 1.
Experiment 2: DARLAM 1×CO2
and 2×CO2 simulations
Over the Australasian region (71°E177°E, 12°N57°S),
DARLAM has been driven at its lateral boundaries by output from the CSIRO
Mark 2 GCM for 20 years of 1×CO2 and 2×CO2
conditions (McGregor et al., submitted). Observed
precipitation and temperature are much better simulated in DARLAM than
in the CSIRO Mark 2 GCM (Figure 4), largely because
DARLAM captures topographic effects not able to be represented in the
GCM. Under 2×CO2 conditions, DARLAM simulated
patterns of precipitation and temperature change which can differ significantly
from those simulated by the host GCM (Whetton et al.,
1997a).
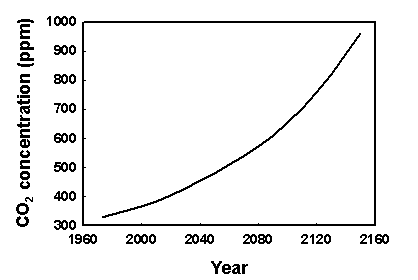
Fig 4. Atmospheric CO2
concentration (parts per million - ppm) used in the CSIRO coupled GCM
for IS92a transient CO2 simulations (from Hirst
et al., 1997).
The climate variables saved are listed in Appendix 2.
In more recent simulations, DARLAM has been run at 60 km resolution over
south-eastern Australia (Whetton et al., 1997a,
b), New Zealand, South Africa and south-east Asia. New simulations
are planned at this resolution over different regions including Queensland
and the south Pacific.
Experiment 3: CSIRO coupled GCM transient CO2
simulation
The coupled model has been driven by the IPCC IS92a CO2
scenario which increases concentrations at a rate of 0.5% per year for
the first 100 years, and slightly faster thereafter (Hirst
et al., 1997, Fig. 4). This scenario represents the IPCCs central
estimate based upon a range of assumptions about future population
and economic growth, and energy supplies in the absence of climate policies
beyond those already adopted. Radiative effects of changes in other greenhouse
trace gases are excluded, but will be included in future experiments.
The reference CO2 concentration is 330 parts
per million (ppm) for the year 1975. After 130 years the CO2
concentration doubles to 660 ppm, and trebles after 180 years. At the
time of 2×CO2, the global mean warming
of near-surface air is 2.2°C, and at 3×2 the warming is 3.8°C
(Fig. 5). This is about half the warming generated
by Experiments 1 and 2, largely due to the greater uptake of heat by the
deep ocean in the coupled model which results in less warming near the
surface.
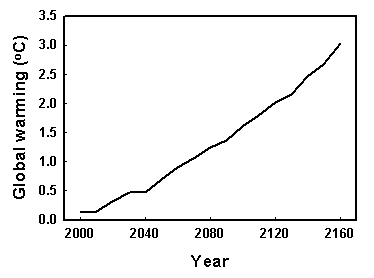
Fig 5. The global mean change (IS92a-1×CO2)
in surface temperature (oC) simulated by the CSIRO coupled GCM (from Hirst,
1996).
The climate variables saved from the coupled GCM experiment are listed
in Appendix 3.
Output available
A wide range of climatic variables have been saved from each experiment
at various time intervals and at various vertical levels. Broad groupings
of the variables include:
- temperature
- precipitation
- wind
- pressure
- cloud
- evaporation
- radiation
- humidity
- soil moisture
- runoff
- snow
- sea-ice
- mixing ratio
- heat flux
In Experiment 1 (CSIRO slab
GCM), for 30 years of both 1×CO2 and
2×CO2 conditions, 38 variables were saved
at 8-hourly intervals, 13 variables were saved 24-hourly, and 95 variables
were saved as monthly averages (Appendix 1).
In Experiment 2 (DARLAM), for
20 years of both 1×CO2 and 2×CO2
conditions, 13 variables were saved at 3-hourly intervals, 51 variables
were saved 12-hourly, 2 variables were saved 24-hourly, and 51 variables
were saved as monthly averages (Appendix 2).
In Experiment 3 (CSIRO coupled GCM),
for 185 years of transient CO2 conditions, 98
atmospheric variables and 108 oceanic variables were saved as monthly
averages (Appendix 3).
Value-added products
Tailored output
The output listed in Appendices 1, 2 and 3 may not suit the applications
of all users. CSIRO is open to negotiation about supplying alternative
products that are tailored to meet specific needs. For example:
- users may want variables which were not saved, but can be derived
from a combination of saved variables (e.g. dewpoint temperature).
- in some cases the model may be re-run to provide different variables
or higher temporal resolution
users may want long-term (multi-year) averages of particular variables,
rather than many years of daily or monthly data
- regionally-specific subsets of the data may suit users with limited
geographical interest or limited data storage facilities
- output in the form of maps or graphs can also be produced
- data scaled for particular years in the future, taking into account
different greenhouse gas emission scenarios, different sulphate aerosol
emission scenarios, different global climatic sensitivities to changes
in greenhouse gas concentrations, and different patterns of climate
change simulated by various climate models (see OzClim
below).
Many other possibilities exist for adding value to output saved from
CSIRO climate change experiments. CSIRO supplied out put to over 40 collaborative
projects from 1990 to 1995 (Hennessy et al., 1995)
and output to a further 20 collaborative projects from 1995-1997 Collaboration
in scenario development and impact projects 1990-97). Where possible,
supply of value-added data will be free of charge, particularly for funded
collaborative projects.
OzClim PC software
CSIRO and the International
Global Change Institute (IGCI), University of Waikato (New Zealand)
have developed a PC-based software package called OzClim which runs under
Microsoft Windows 95. OzClim enables regional scenarios of climate change
to be generated for the whole or selected parts of Australia at various
spatial resolutions, for any date between 1990 and 2100 (Jones,
1996; Collaboration in scenario development and impact projects 1990-97).
The user can select from a range of greenhouse gas emission scenarios,
global climate sensitivity assumptions, and GCM or RCM patterns of climate
change.
The advantage of this approach is that the effect of a range of options,
assumptions or policy measures can be explored in an internally consistent
way, and the effects of uncertainty can be calculated explicitly. The
system will also allow easy updating as new scenario information becomes
available. This will be necessary as sulfate aerosol and ozone depletion
effects are incorporated into climate models. Output is displayed in the
form of colour maps and graphs (Fig 6).
It is planned to couple OzClim with a GIS database, and with various
impact models for sectors such as agriculture, ecosystems and hydrology.
The integrated system will also be capable of use for climate variability
studies using historical climate data sets.
Disclaimer
Obtaining output
The first point of contact regarding access to CSIRO climate change output
is through the Climate Impact Liaison Officer, Dr Roger Jones, at CSIRO
Division of Atmospheric Research. Output can be provided in a range of
formats or grid resolutions to suit different applications, and can be
supplied on Exabyte / DAT media or transferred between computers via FTP.
As part of the Climate Change Research Program, the Climate Change Impacts
and Adaptation Project also co-ordinates a great deal of information on
climate change impacts research in Australia. This is available on request
through Dr Jones.
Inquiries about specific aspects of the climate change experiments, information
about forthcoming experiments, requests for alternative variables to be
saved in new experiments, or requests for material cited in this report
can also be directed through Dr Jones, whose contact details are:
Dr Roger Jones
CSIRO Division of Atmospheric Research
Private Bag No. 1
Aspendale, Victoria, Australia, 3195
Ph +61 3 9239 4555
Fax +61 3 9239 4444
E-mail roger.jones@csiro.au
Appendix 1
CSIRO9 Mark 2 global climate model output
1×CO2 & 2×CO2
experiments
30 year timeseries
(350 km × 625 km resolution over the globe)
Data for 9 atmospheric sigma levels (L×surface pressure,
where L = 0.979, 0.914, 0.803, 0.670, 0.500, 0.340, 0.197, 0.086 and 0.021)
8-hourly data:
- Moisture mixing ratio
- Pressure at surface
- Temperature
- Temperature at surface
- Wind speed: meridional (south>north)
- Wind speed: zonal (west>east)
24-hourly data
(* = average of 48 half-hourly values):
- Cloud fraction: total *
- Evaporation: potential and actual *
- Precipitation: convective and *
- Pressure: sea-level
- Radiation: downward shortwave at surface *
- Radiation: net longwave at top of atmosphere *
- Relative humidity at 2m above surface *
- Soil moisture in lowest layer (28.5278.5 cm below surface)
- Soil moisture in middle layer (328.5 cm below surface)
- Temperature at 2m above surface *
- Temperature at surface *
- Temperature: max & min at 2m above surface
- Wind speed at 10m above surface *
Monthly-mean data
- Albedo
- Cloud fraction: low level, middle level, high level, total
- Evaporation: potential and actual
- Heat flux: latent
- Heat flux: sensible at surface
- Ice concentration
- Ice-ocean heat flux
- Ice-ocean salt flux
- Moisture mixing ratio
- Precipitation: total, convective, canopy interception
- Pressure: sea-level
- Radiation: clear sky longwave out at top of atmosphere
- Radiation: clear sky net longwave at surface
- Radiation: clear sky net shortwave at surface
- Radiation: clear sky shortwave out at top of atmosphere
- Radiation: downward longwave at surface
- Radiation: downward shortwave at surface
- Radiation: longwave out at top of atmosphere
- Radiation: net longwave at surface
- Radiation: net shortwave at surface
- Radiation: shortwave out at top of atmosphere
- Relative humidity at 2m above surface
- Runoff
- Sea-ice depth
- Snow depth
- Soil moisture in lowest layer (28.5278.5 cm below surface)
- Soil moisture in middle layer (3.028.5 cm below surface)
- Temperature
- Temperature: max, min, mean at surface (bare soil)
- Temperature: max, min, mean at surface (vegetated)
- Temperature of lowest soil layer (28.5278.5 cm below surface)
- Temperature of middle soil layer (3.028.5 cm below surface)
- Temperature: extreme monthly max and min at 2m above surface
- Temperature: max, min, average at 2m above surface for bare ground
and canopy
- Wind speed at surface
- Wind speed: meridional (south>north)
- Wind speed: zonal (west>east)
- Wind stress: meridional (south>north) at surface
- Wind stress: zonal (west>east) at surface
Note
Other variables not listed here and timeseries-averages can be derived
from archived data upon request.
Appendix 2
CSIRO regional climate model (DARLAM) output
1×CO2 & 2×CO2
experiments
10 year timeseries
(125 km resolution over Australasia (71 °E177 °E, 12 °N57
°S))
Data for 9 atmospheric sigma levels (L×surface pressure,
where L = 0.979, 0.914, 0.803, 0.670, 0.500, 0.340, 0.197, 0.086 and 0.021)
3-hourly data:
- Cloud fraction: total
- Heat flux: latent at surface
- Heat flux: sensible at surface
- Radiation: clear sky net shortwave at surface
- Radiation: downward longwave at surface
- Radiation: net shortwave at ground
- Radiation: shortwave at top of atmosphere
- Relative humidity at 2m above surface
- Temperature at surface
- Temperature at 2m above surface
- Wind speed at 2m above surface
- Wind speed at 3m above surface
- Wind speed at 10m above surface
12-hourly data:
- Cloud fraction: low, middle, high
- Moisture mixing ratio
- Precipitation: total, convective
- Pressure at surface
- Radiation: clear sky longwave at top of atmosphere
- Radiation: long wave at top of atmosphere
- Runoff
- Soil moisture in lower layer (0100 cm below surface)
- Temperature
- Temperature at surface
- Temperature of middle soil layer (3.028.5 cm below surface)
- Temperature of lowest soil layer (28.5278.5 cm below surface)
- Wind speed: meridional (south>north)
- Wind speed: zonal (west>east)
- Wind stress: meridional (south>north) at surface
- Wind stress: zonal (west>east) at surface
24-hourly data:
- Temperature: max & min at 2m above surface
Monthly-mean data:
- Cloud fraction: low, middle, high
- Moisture mixing ratio
- Precipitation: total, convective
- Pressure at surface
- Radiation: clear sky longwave at top of atmosphere
- Radiation: long wave at top of atmosphere
- Runoff
- Soil moisture in lower layer (0100 cm below surface)
- Temperature
- Temperature at surface
- Temperature of middle soil layer (3.028.5 cm below surface)
- Temperature of lowest soil layer (28.5278.5 cm below surface)
- Wind speed: meridional (south>north)
- Wind speed: zonal (west>east)
- Wind stress: meridional (south>north) at surface
- Wind stress: zonal (west>east) at surface
Note
Other variables not listed here and timeseries-averages can be derived
from archived data upon request.
Appendix 3
CSIRO9 global coupled ocean-atmosphere climate model output
IPCC IS92a CO2 experiment
185 year timeseries
(350 km × 625 km resolution over the globe)
Data for 9 atmospheric sigma levels (L×surface pressure,
where L = 0.979, 0.914, 0.803, 0.670, 0.500, 0.340, 0.197, 0.086 and 0.021)
ß Data for 21 oceanic levels at depths of 12.5, 37.5, 65, 98.5,
138.5, 185, 240, 310, 410, 545, 710, 905, 1130, 1395, 1720, 2125, 2575,
3025, 3475, 3925, 4375 metres below surface
Monthly-mean data
- Albedo
- Cloud fraction: low level, middle level, high level, total
- Evaporation: potential and actual
- Heat flux: latent
- Heat flux: sensible at surface
- Freshwater flux at ocean surface
- Ice concentration
- Ice-ocean heat flux
- Ice-ocean salt flux
- Moisture mixing ratio
- Ocean current velocity: meridional (south>north)ß
- Ocean current velocity: zonal (west>east) ß
- Ocean current velocity: vertical ß
- Oceanic streamfunction ß
- Precipitation: total, convective, canopy interception
- Pressure: sea-level
- Radiation: clear sky longwave out at top of atmosphere
- Radiation: clear sky net longwave at surface
- Radiation: clear sky net shortwave at surface
- Radiation: clear sky shortwave out at top of atmosphere
- Radiation: downward longwave at surface
- Radiation: downward shortwave at surface
- Radiation: longwave out at top of atmosphere
- Radiation: net longwave at surface
- Radiation: net shortwave at surface
- Radiation: shortwave out at top of atmosphere
- Relative humidity at 2m above surface
- Runoff
- Salinity ß
- Sea-ice depth, west>east velocity, south>north velocity
- Snow depth
- Soil moisture in upper layer (3.028.5 cm below surface)
- Soil moisture in lower layer (28.5278.5 cm below surface)
- Soil percolation
- Temperature ,ß
- Temperature: max, min, mean at surface (bare soil)
- Temperature: max, min, mean at surface (vegetated)
- Temperature of middle soil layer (3.028.5 cm below surface)
- Temperature of lowest soil layer (28.5278.5 cm below surface)
- Temperature: extreme monthly max and min at 2m above surface
- Temperature: max, min, average at 2m above surface for bare
- ground and canopy
- Wind speed at surface
- Wind speed: meridional (south>north)
- Wind speed: zonal (west>east)
- Wind stress: meridional (south>north) at surface
- Wind stress: zonal (west>east) at surface
Note
Other variables not listed here and timeseries-averages can be derived
from archived data upon request.
References
CSIRO (1996): Climate change scenarios for Australia.
CSIRO Division of Atmospheric Research, 8 pp.
Dix, M.R. and Hunt, B.G. (1995): Climatic modelling
doubling of CO2 levels and beyond. Final
report to the Federal Department of the Environment, Sport and Territories.
CSIRO Division of Atmospheric Research, 28 pp.
England, M.H. (1995): Using chlorofluorocarbons to
assess ocean climate models, Geophys. Res. Lett., 22 (22), 30513054.
Gordon, H.B. and O'Farrell, S.P. (1997) Transient
climate change in the CSIRO coupled model with dynamic sea ice. Monthly
Waether Review, 125(5), 875907.
Hennessy, K.J., Whetton, P.H. and Pittock, A.B. (1995)
CSIRO Climate Change Research Program: Collaboration in Scenario Development
and Impact Projects 19901995. CSIRO Division of Atmospheric Research
Report, 51 pp.
Hirst, A.C., Gordon, H.B. and OFarrell, S.P.
(1997): Response of a coupled ocean-atmosphere model including oceanic
eddy-induced advection to anthropogenic CO2
increase. Geophys. Res. Lett., 23(23), 33613364.
Houghton, J.T., Meira Filho, L.G., Callander, B.A.,
Harris, N., Kattenberg, A. and Maskell, K. (eds.), Climate Change 1995,
The Science of Climate Change. Contribution of Working Group 1 to the
Second Assessment Report of the IPCC, Cambridge University Press, 572
pp.
Jones, R.N. (1996): OzClim A Climate Scenario
Generator and Impacts Package for Australia. CSIRO Division of Atmospheric
Research.
Kattenberg, A, Giorgi, F., Grassl, H., Meehl, G.A.,
Mitchell, J.F.B., Stouffer, R.J., Tokioka, T., Weaver, A.J. and Wigley,
T.M.L. (1996): Climate models projections of future climate. In:
J.T. Houghton, L.G. Meira Filho, B.A. Callander, N. Harris, A. Kattenberg,
and K. Maskell (eds.), Climate Change 1995, The Science of Climate Change.
Contribution of Working Group 1 to the Second Assessment Report of the
IPCC, Cambridge University Press, 285358.
Kowalczyk, E.A., Garratt, J.G. and Krummel, P.B. (1994):
Implementation of a soil-canopy scheme into the CSIRO GCM regional
aspects of the model response. Technical Paper 32, CSIRO Division of Atmospheric
Research.
McGregor, J.L. (1993): Economical determination of
departure points for semi-Lagrangian models. Mon. Wea. Rev., 121, 221230.
McGregor, J.L., Walsh, K.J. and Katzfey, J.J. (1993):
Nested modelling for regional climate studies. In: A.J. Jakeman, M.B.
Beck and M.J. McAleer (eds.), Modelling Change in Environmental Systems,
J. Wiley and Sons, 367386.
McGregor, J.L., Katzfey, J.J. and Nguyen, K.C. (submitted):
Seasonally-varying nested climate simulations over the Australian region.
J. Climate.
OFarrell, S.P. (1998): Investigation of the
dynamic sea-ice component of a coupled atmosphere sea-ice general circulation
model. J. Geophys. Res. (in press)
Walsh, K.J. and McGregor, J.L. (1995): January and
July climate simulations over the Australian region using a limited-area
model. J. Climate, 8 (10), 23872403.
Watterson I.G., OFarrell, S.P. and Dix M.R.
(1997): Energy transport in climates simulated by a GCM which includes
dynamic sea-ice. J. Geophys. Res., STRONG>102(D10), 1102711037.
Whetton, P.H., Katzfey, J.J., Nguyen, K., McGregor,
J.L., Page, C.M., Elliot, T.I. and Hennessy, K.J. (1997a): Fine Resolution
Climate Change Scenarios for New South Wales - Part 2: Climate Variability
CSIRO 1996-1997 Consultancy Report for NSW Environment Protection Authority,
51 pp.
Whetton, P.H., Wu, X., McGregor, J.L., Katzfey, J.J.
and Nguyen, K. (1997b): Fine Resolution Assessment of Enhanced Greenhouse
Climate Change in Victoria. CSIRO Consultancy Report. Victorian Environment
Protection Authority Publication 574, 34 pp.
Acknowledgments
The climate model data resources listed in this report have been made
available by the efforts of many climate modellers and data analysts in
CSIRO Climate Change Research Program.
Funding for the generation and analysis of CSIRO climate change data
is provided by the National Greenhouse Research Program via the Federal
Department of the Environment, Sport and Territories, the Governments
of Victoria, New South Wales, Queensland, the Northern Territory and Western
Australia, and CSIRO.
Comments from Dr Chris Mitchell, Dr Barrie Pittock and Dr Roger Jones
of CSIRO Division of Atmospheric Research were very useful.
Data listings from Mr Martin Dix and Dr Jack Katzfey of CSIRO Division
of Atmospheric Research are particularly appreciated.
This work is a product of the CSIRO Climate Change Research Program.
The document was written by Kevin Hennessy and the electronic version
compiled by Roger Jones. Back to Climate Impact Group |